Have you worked with fruit flies in science class or spotted these very small insects flying around ripe bananas or other fruit? Imagine the size of a fruit fly’s brain. How would you measure something that small? And how might experimenting with the chemicals in brains that small help human beings work towards understanding and one day finding a cure for diseases like Parkinson’s?
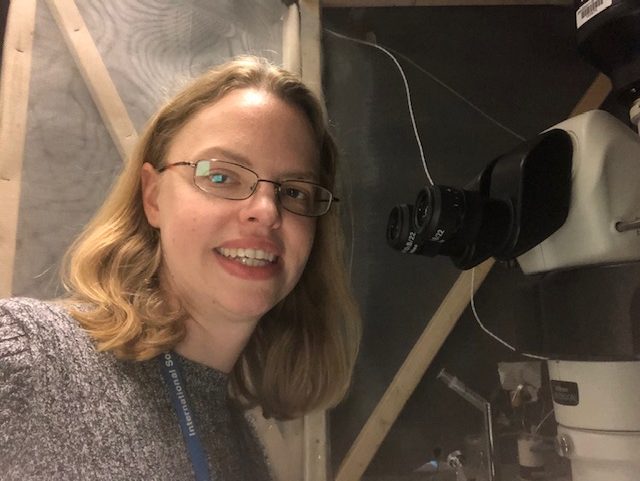
A nanometer (nm) is a tiny piece of a meter. (Spread your arms wide and look at the distance between your left and right hands. That’s about a meter or, if you’re adult-sized, a bit more.) Split a meter into a billion pieces of equal length and each one of those pieces is a nanometer long. A nanometer is a millionth of a millimeter.
Look at a meter stick or a ruler that splits centimeters into tenths, which are millimeters. Millimeters are quite small, but you can see things that are a millimeter wide. A fruit fly’s brain, for instance, is a bit smaller than a poppy seed: tiny, but you can see it. You can’t see things that are a nanometer or even many nanometers long: they’re microscopic.
Neurochemist Jill Venton, an analytical chemist, and her colleagues create sensors — tools so small that they’re measured in nanometers. Each sensor’s base is shaped like a ball. It’s “a sphere but we put all these spiky cones around it.” Then they put these tools into the brains of fruit flies (Drosophila melanogaster). Building these sensors — microscopic electrodes — involves very precise measurement and geometry skills.
“We print these tiny things on a wire: a 25 micron-wide wire. (A micron is a millionth of a meter … a thousandth of a millimeter (mm). How many of Venton’s wires would fit into one mm?) And then we take that wire back to our lab and put them [the electrodes] in the brain to measure neurotransmitters.” Neurotransmitters like dopamine, serotonin, and acetylcholine are chemicals which the cells of our nervous system (neurons) use to communicate with each other and with muscles.
The printer Venton and her colleagues use — a Nanoscribe, which uses 2-photon lithography to create tiny structures — is at Oak Ridge National Laboratory, a Tennessee lab sponsored by the U.S. Department of Energy. It contains liquid that can form polymers (solid plastic). The machine releases two beams of light and makes polymers only in spots the light beams hit. This system’s precision allows the Nanoscribe to build things that are as small as 500 nm wide — what Venton calls “3D printing on a tiny, tiny scale.”
“I don’t have a great love of geometry, I have to admit, but we have to use our knowledge of geometry to 3D-print a structure. You have to be able to draw it to print.” And because three-dimensional printers create things from the bottom up, “you can’t go back and print underneath something.” You have to think carefully about the shape of the device you’re creating and ask yourself “How do I design it so I’m only printing on the top?”
At first, Venton’s team of scientists tried making electrodes with tiny cylinders sticking out of them. But they found that the cylinders weren’t as stable as cones, so they changed from cylinders to cone-shaped spikes. The spikes create extra surface area — more than just a sphere would have. This means that “the electrode can measure more neurons when I put it into a brain.”
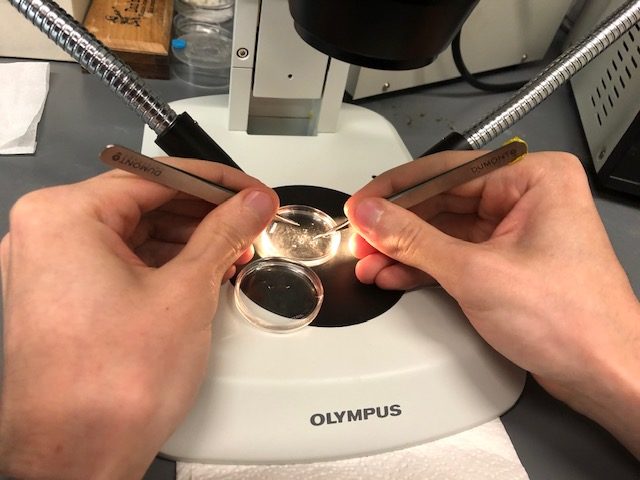
“Fruit flies have brains just like you,” says Venton. Her team uses their sensors to measure neurotransmitters such as dopamine and serotonin in fruit fly brains. When people have Parkinson’s disease, “the biggest problem is that your dopamine neurons die off,” affecting your ability to move. “Humans who get Parkinson’s have trouble walking or moving. Flies have the same thing.”
Venton’s team puts fruit flies into an activity monitor. Laser beams in the monitor keep track of how much the flies move. The scientists “count laser beam crosses as a behavioral measure.” Groups of flies might cross the laser beams 100 times in a certain period of time. A group of flies with Parkinson’s, on the other hand, might move across the beams just 50 times. Keeping track of all of this does not just involve “counting but involves a bunch of statistics.” You “can’t just look at the numbers themselves because there will be natural variation in the animals.” Like individual humans, some healthy flies move around more or less than others do. Venton and her colleagues use statistics to measure the probability that their samples demonstrate a relationship between activity levels and Parkinson’s.
Then they put electrodes (the sensors they’ve designed) into the flies’ brains to measure the amount of dopamine there. They track this as the disease affects each group of flies more and as it ages. Their work aims to help us better understand the causes of Parkinson’s and to test drugs designed to help the brains of people who have it. “We’ll never solve Parkinson’s in the fruit fly but we can learn how these drugs work and hopefully [explore] some of the causes of Parkinson’s” and “apply that to humans in the future.”
Another advantage of Venton’s work is that “any of the sensor designs we come up with … can be used to sense anything. Other scientists “could use this stuff — these electrochemical sensors” to find and measure pollutants in water or for other important applications.
As a child, Jill considered herself lucky because “my dad was a scientist.” He helped her with her math homework. “I’d sit around in college and ask him calculus questions and he’d say ‘Oh, I haven’t done this in forever … but then he could figure it out.’” And she and her sister built things with their father’s molecular model kits. “We played with them like they were Tinkertoys.”
Jill father’s work as a chemist didn’t just help her learn math and to have fun building molecular models. He worked in Washington D.C. at Gallaudet, a university whose mission is to educate students who are deaf or hard-of-hearing. When she visited his lab, Jill set up test tubes, beakers, and other glassware for that day’s experiments. And she helped students learn how to use equipment, measure liquids by the drop, and test different substances’ pH.
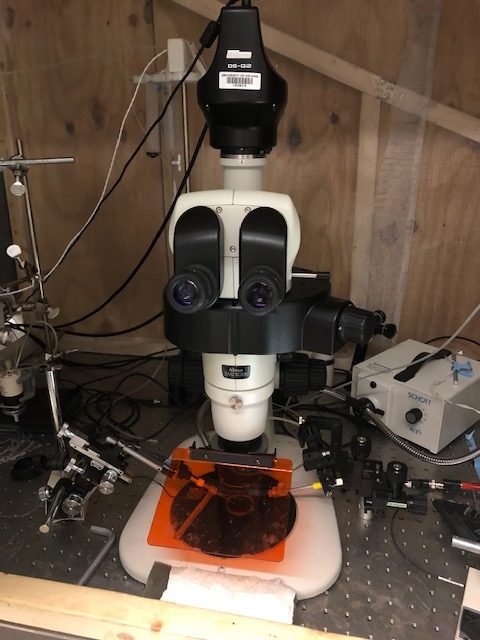
Knowing some sign language helped Jill communicate with her father’s students. Working with them “gave me a great appreciation that all kinds of people can do science.”
But what kind of science would she choose for herself? Her high school biology courses focused on memorizing facts. As a result, “biology just seemed solved to me.” Chemistry, on the other hand, involved working through problems, more like math. “So I went into chemistry and it wasn’t really until my junior or senior year in college that I saw the amazing things that people were doing on the border between chemistry and biology, using their tools to measure different biological compounds.” Venton realized that she could apply what she had learned as a chemist to biology.
Math has helped her every step of the way. In her lab, “we still use a lot of what I call basic math. I don’t do calculus every day but man do I use a lot of multiplication, division, unit conversions, and scientific notation.” It’s “extremely important,” to understand how to do these types of math and to do them well. “This is the kind of math you’ll have to do every day if you have a job as an analytical chemist.”
Venton told Math4Science about a laboratory investigating the Zika virus that was shut down. Their testing proved to be wrong due so a simple math error on a spreadsheet: the concentration of Zika was calculated incorrectly. Says Venton, “it’s important to solve your math equations!”
Writing’s also important for her work. “We spend a lot of time writing about [the experiments that] we did…. If I can’t write about it well, other people won’t know” what she and her colleagues discovered. Venton loves to write and to help her students improve their writing skills. She tells her students at the University of Virginia “Hey guys, this is a story. How can we make it more exciting? How can we write it more clearly? How can we use the data that we get to tell a story?”
Connecting writing, math, biology, and chemistry, Venton and her colleagues help us better understand chemical processes and “see” what’s happening in tiny, microscopic spaces like those of the brains of fruit flies.
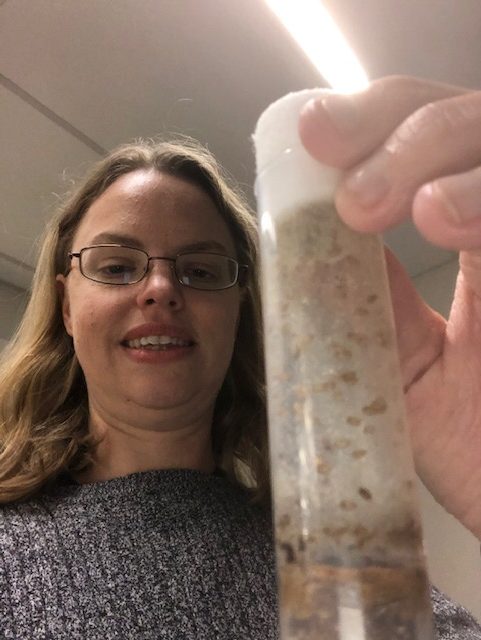