“It will soon be possible to change your DNA not just for medical reasons, but at will — should that be legal?” ask Molecular and Cell Biology Professors Fyodor Urnov and Jasper Rine in the description of “Biology for Voters,” the course they teach together at the University of California at Berkeley. Genetic engineering, making changes in animals’ (including humans’) DNA, raises ethical questions, like the ones Urnov and Rine explore in their class. But in the field of biomedicine its value is becoming quite clear.
DNA, a beautiful double helix of genes inside our cells, contains a code that guides the growth of each living being on Earth. Genetic Engineer Fyodor Urnov helps figure out how to make changes in DNA, changes which fix problems. “My work is in the field of developing new approaches to treat human disease.” Urnov and his colleagues at Sangamo BioSciences search for and test treatments for illnesses of the blood, including sickle cell anemia, AIDS, hemophilia, and thalassemia (a condition that involves not being able to make enough red blood cells and causes anemia). And they also focus on finding ways of treating diseases of the nervous system, such as Parkinson’s and Huntington’s, which killed the singer-songwriter Woody Guthrie.
There are sicknesses that arise within the larger systems of our bodies, problems such as heart attacks, which happen when blood vessels are clogged and cannot deliver enough oxygen to the heart. “In contrast,” Urnov explains, “a [genetic] disease like sickle cell disease is an illness of the cell: an individual cell cannot make enough proper hemoglobin. If we can fix the cell, we can fix the disease.”
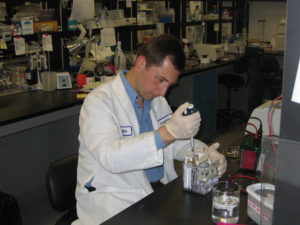
Because “everything that has to do with cells is 1,000 times smaller than a grain of sand,” Urnov has to use tiny, tiny tools. “To work with things inside the living cell, you need to talk not about inches, feet, or millimeters, but nanometers.” (A nanometer is one billionth of a meter. Look at the lines drawn very close together on a meter stick: the space between two of those lines is one millimeter. A nanometer is a millionth of a millimeter, too small to see without a microscope.)
After many years of struggling to find a way to make genetic changes in cells, scientists are now able to “walk up to a living cell with a nanomachine, enter the nucleus of that cell,” identify one particular gene, and fix the problem that gene is causing. What is the nanomachine that Urnov uses? “It’s a protein: a little molecular agent, a tiny machine” containing parts like the blades of scissors. “The nanomachine that we build out of protein has two blades and the ability to ‘see’ a specific gene,” cut it, and repair it.
DNA and RNA, which carries out DNA’s instructions, are each written in code (if you have studied genetics in biology class, you probably came across the names of the individual units in nucleic acids which make up these codes: cytosine, thymine [this one’s only in DNA], uracil [this one’s only in RNA], adenine, and guanine). Each part of this code, each unit in a nucleic acid, is so small that you need nanometers or angstroms, which are even smaller than nanometers, to measure its size. Each stretch of a nucleic acid (each gene) contains “little protrusions that are unique to it.” The protein-nanomachine that Urnov uses, called a “zinc finger,” can read these bumps and indentations, much like a blind person uses her or his fingers to read Braille. The protein’s “little fingers, made out of chemicals … run down the DNA to read it until they find the text that is interesting to them.”
Using zinc fingers and other tiny tools, Urnov and his colleagues are able to “take specific kinds of cells out of the body, genetically fix them, and put them back in.” This type of genetic engineering “works well with blood cells.” Scientists take a sample of cells, and treat them with zinc fingers, repairing their DNA. They then grow more of these healthier cells in a very sophisticated version of a Petri dish and return them to the person they came from. “The room where this genetic engineering happens has to have incredibly rigorous controls for air purity, to filter out [even] the most minute particle. Anything larger than 1/10,000 of a meter is filtered out, to keep the cells germ-free,” Urnov explains.
How did Urnov first become interested in science? “I received a microscope as a birthday present at age 12.” He remembers this as if it were yesterday. “Until then,” he wanted to be a journalist or “a cosmonaut, like Yuri Gregarin,” the first human being to travel into outer space. “There was an ant crawling across my desk and I put that ant just under the microscope and it sat there. I looked at the eye of an insect.” Those eyes are not like ours: insects have compound eyes, containing “thousands of little light-sensing units,” which “mesmerized” Fyodor. He also admired the “beautiful joints of the ant’s antennae. I remember gasping” at the structure within “this tiny living creature.” “I fell for life: I fell for biology right there and then.”
In Russia, where Fyodor lived as a child, “math was taught abstractly: the area of a circle is pi r squared and that’s it.” He contrasts this formulaic, practical approach to teaching math to the more playful, yet sometimes inefficient, ways math is taught in some U.S. schools. “Where’s the Venn diagram between the abstract beauty of math and the applicability?” “There’s a Calvin and Hobbes strip in which Calvin, who hates math, says to the tiger ‘I don’t know how to solve this problem, but I have to.’ And Hobbes says, ‘In math, you have to call the answer y, as in … why do we care?’”
How to get students to care about math is a problem Urnov thinks a great deal about, a problem that matters very much to his field of science. “Any practicing geneticist must be fundamentally math and probability savvy,” says Urnov. He asks us to imagine treating four people for a rare disease. If two of them get better and two of them do not, “How can you tell if you’ve made a difference? Is this effect real or a placebo?” Should you continue testing that treatment or stop? Understanding probability and knowing how to use it to analyze the results of their research help Urnov and his colleagues make these kinds of decisions.
Another example of the importance of math is in genetic medicine. Says Urnov, perhaps a doctor tells Angelina Jolie or another patient that women in her family have a 60% chance of developing breast cancer. “What does the 60% mean?” A doctor needs to walk the patient through these numbers, helping them understand them … and to be able to do that, “you have to study some math.” In this case, the percentage might mean that a woman has a 60% chance of getting cancer at some point during her lifetime. But perhaps her chance of actually dying of cancer is only a three to five percent chance. One kind of cancer is “quite curable if detected early” and women who get it have a 90% chance of surviving. But another, genetic type of cancer is much more aggressive: if a woman gets that, her chances of surviving might be much lower. In other words, a doctor might have to explain to a woman the difference between very different types of numbers and what those numbers might mean for her future.
Urnov majored in biology at Moscow State University, where he “spent an unbelievably wonderful time in labs and in classes, doing field work.” He enjoyed working with “cool gadgets” like microscopes in biology labs. And he “loved the fact” that everything he was learning “could be used to better understand how living things work, for instance how insects” end up with specialized eyes and “why only female cats become calico.”
“This beauty had numbers in it.” “It helped greatly that I always loved math: the beauty of equations, how they are so … there’s an absolute truth to arithmetic, calculus, trigonometry. A certain principle that is always true makes you feel omnipotent.” That feeling of accomplishment must still be familiar to Urnov, as he helps his students understand math and science and uses his own knowledge of those subjects to find cures for diseases.